Posted: December 1, 2020
Animal pollinators provide an essential service to flowering plants, worldwide.
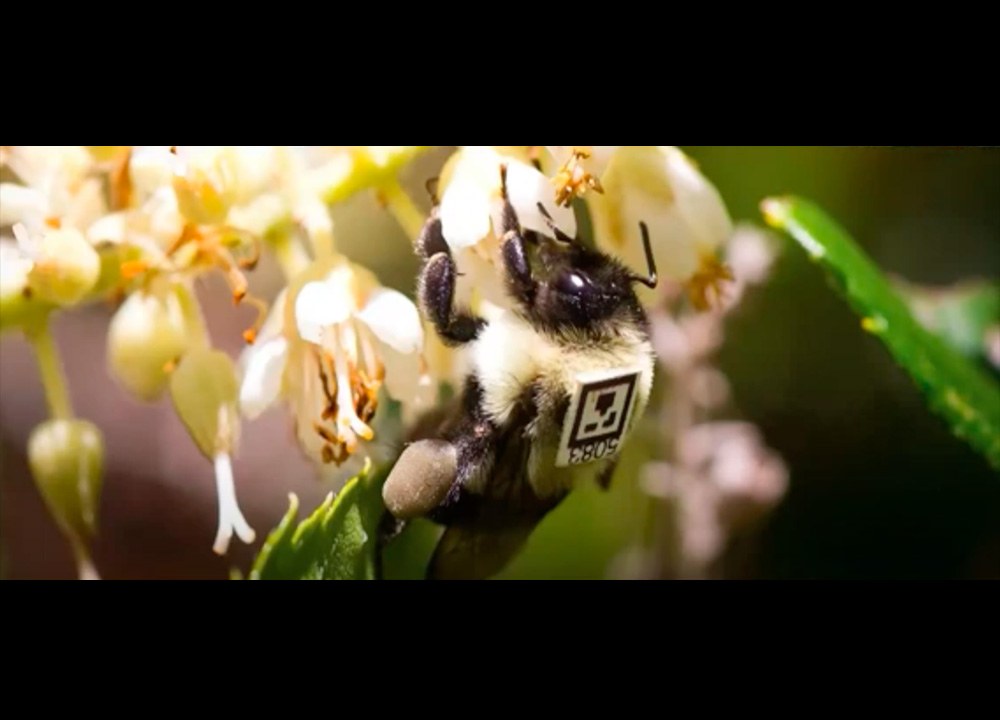
Indeed, 308,000 flowering plant species (88% of the global diversity) are visited by pollinators [1]. These animals provide ecosystem services central to our modern diet: Roughly one-third of the global food supply and 75% of all agricultural crops depend on pollination, including many of the fruits, vegetables, nuts, and seeds we eat everyday [2]. Concurrently, human activities, such as the application of pesticides, habitat fragmentation, and climate change are altering the environment and adversely impacting the health, diversity, and abundance of pollinators [3]. From a growing body of research, we are now learning that human-mediated stressors don't affect all pollinators in the same way, and it can be challenging to predict, measure or mitigate some of the resultant changes we observe in global pollinator populations. Oftentimes, pollinator responses to stress are 'hidden' and, while difficult to detect, may have long-lasting, detrimental impacts to their health in a given landscape.
James D. Crall – currently a USDA-NIFA Postdoctoral Fellow at Harvard University working with Benjamin de Bivort – studies these "hidden" effects of a widely used pesticide (known as neonicotinoid insecticides, or 'neonics') on social insect societies. Neonics work by targeting the nervous system in insects, and are known to be widespread and persistent in the environment [4]. A recent study demonstrated that 75% of global honey supply samples contained detectable levels of neonicotinoids [5].
In contrast to studies of acute and lethal exposure to insecticides, of particular interest to James are their sublethal effects. 'Sublethal effects' include nonlethal responses to pesticide exposure that result in biological, demographic, or behavioral changes to a population or individual. "It's pretty clear that sublethal effects [of insecticides] can impair colony growth and success, and even have trophic cascading effects, for example, on groundwater and fisheries." James work highlights three big challenges in understanding how sublethal effects impact insect behavior. The initial challenge is understanding the direct impact that agrochemicals have on an individual or population. Then, we can consider how these effects interact with other stressors, such as air temperature and other abiotic conditions, nutrition, etc. Finally, do these effects vary within or between species?
Most studies evaluating sublethal effects in response to chemical exposure feature social pollinators such as the European honey bee (Apis mellifera) and the common eastern bumble bee (Bombus impatiens). Speaking over a video clip of a bumble bee navigating a field of flowers, James points out the scope of the complexity of this behavior given such a “spatially complex and temporally dynamic environment, and a brain which is orders of magnitude smaller than the human brain… arguably outperforming any currently operating human made drone." This is the life of pollinators that we humans are typically most familiar with, but they also exhibit complex and understudied behaviors within their nests as well. Bumble bees in particular offer an excellent opportunity to study the impacts of stressors on this hidden world [6]. "Bumblebees are great because they come in a box from the internet" James explains.
With colleagues, James' research has led to the creation of the 'BEEtag', a physical tag-based tracking system, where individual bees are fitted with a QR-code like sticker on their thorax. These individual tags, in turn, are detected and monitored by a computer hooked up to a video camera [8]. With this tool, James is able to continuously monitor what every bee is doing within a colony all at once and over time. Studies using this tool have revealed an enormous amount of variation in individual behaviors. For example, James has found that individual workers occupy different parts of the nest within the colony and over time – which allows us to better predict how colonies experience and react to stressors [9]. Some of his experiments using BEEtag have already revealed that at high doses, imidacloprid (a commonly applied neonicotinoid insecticide) negatively impacts nursing behavior and overall activity of workers in the colony, leading to fewer social interactions among workers [7]. And more recently, James and his colleagues have built a robot which, paired with BEEtag, monitors the behavior of multiple individuals within multiple colonies at once [10]. One of the insights to emerge from linking this new tool with colony observation was that bumblebees respond to chemical exposure differently at night than they do during the day. More specifically, colonies exposed to imidacloprid may look normal during the day, but experience the worst symptoms at night. Expanding on this insight, James and colleagues have further looked at thermoregulation in bumblebee colonies. Using the same computer monitoring system, James and colleagues found that imidacloprid exposure negatively impacts the ability of species like the bumblebee to maintain a constant, colony-wide "body temperature," which is necessary for raising healthy, immature brood [7]. James found that imidacloprid, in part due to reducing social interactions, causes the colony to run a "lower body temperature" at night than normal, and reduces its ability to recover when the outside temperature goes back up during the day. In a more recent experiment, James found similar results using wild caught bumblebee queens [11], and revealed that colony size might help to buffer the negative impact that imidacloprid has on social thermoregulation.
James' conclusions corroborate a large and growing body of research that shows pesticide exposure can interact synergistically with secondary stressors like changes in temperature or other pesticides [12]. With new and continually improving technological innovations, such as BEEtag, it is becoming easier to measure how social colonies react on fine temporal and/or individual scales. This research can help to inform us of how bees may be impacted by human-mediated environmental change, and improve awareness of which practices we can adopt to ensure their health and safety through a thoughtful and informed integrated pest and pollinator management approach.
For more details on James' and colleagues work, see his talk given for the Penn State University "Global Perspectives in Integrated Pest and Pollinator Management" seminar series.
Sean Bresnahan | MCIBS | Grozinger and Axtell labs
References
[1] Winfree, R., Bartomeus, I., Cariveau, D. P. (2011). Native pollinators in anthropogenic habitats. Annual Review of Ecology, Evolution, and Systematics. 42: 1-22
[2] Aizen, M. A., Garibaldi, L. A., Cunningham, S. A., Klein, A. M. (2009). How much does agriculture depend on pollinators? Lessons from long-term trends in crop production. Annals of Botany. 103(9): 1579-1588
[3] López-Uribe, M. M., Ricigliano, V. A., Simone-Finstrom, M. (2020). Defining pollinator health: a holistic approach based on ecological, genetic, and physiological factors. Annual Review of Animal Biosciences. 8: 269-294
[4] Wood, T. J., Goulson, D. (2017). The environmental risks of neonicotinoid pesticides: a review of the evidence post 2013. Environ Sci Pollut Res Int. 24(21): 17285-17325
[5] Mitchell, E. A. D., Mulhauser, B., Mulot, M., Mutabazi, A., Glauser, G., Aebi, A. (2017). A worldwide survey of neonicotinoids in honey. Science. 358(6359): 109-111
[6] Smith, D. B., Arce, A. N., Rodrigues, A. R., Bischoff, P. H., Burris, D., Ahmed, F., Gill, R. J. (2020). Insecticide exposure during brood or early-adult development reduces brain growth and impairs adult learning in bumblebees. Proc Royal Soc B. 287: 20192442
[7] Crall, J. D., Switzer, C. M., Oppenheimer, R. L., Versypt, A. N. F., Dey, B., Brown, A., Eyster, M., Guérin, C., Pierce, N. E., Combes, S. A., de Bivort, B. L. (2018). Neonicotinoid exposure disrupts bumblebee nest behavior, social networks, and thermoregulation. Science. 362(6415): 683-686
[8] Crall, J. D., Gravish, N., Mountcastle, A. M., Combes, S. A. (2015). BEEtag: A low-cost, image-based tracking system for the study of animal behavior and locomotion. PLoS One. 10(9): e0136487
[9] Crall, J. D., Gravish, N., Mountcastle, A. M., Kocher, S. D., Oppenheimer, R. L., Pierce, N. E., Combes, S. A. (2018). Spatial fidelity of workers predicts collective response to disturbance in a social insect. Nature Communications. 9: 1201
[10] Alisch, T., Crall, J. D., Kao, A. B., Zucker, D., de Bivort, B. L. (2018). MAPLE (modular automated platform for large-scale experiments), a robot for integrated organism-handling and phenotyping. eLife. 7: e37166
[11] Crall, J. D., de Bivort, B. L., Dey, B., Versypt, A. N. F. (2019). Social buffering of pesticides in bumblebees: agent-based modeling of the effects of colony size and neonicotinoid exposure on behavior within nests. Front. Ecol. Evol. 7: 51
[12] Manjon, C., Troczka, B. J., Zaworra, M., Beadle, K., Randall, E. et al. (2018). Unravelling the molecular determinants of bee sensitivity to neonicotinoid insecticides. Curr. Biol. 28(7): 1137-1143