The Center for Pollinator Research
The Center for Pollinator Research
Advancing Pollinator Health and Conservation
+80%
of plants use pollinators to reproduce
1 of 3
bites of food we eat exists because of pollinators
3/4
production of 3/4 of the world's major food crops benefit from pollinators
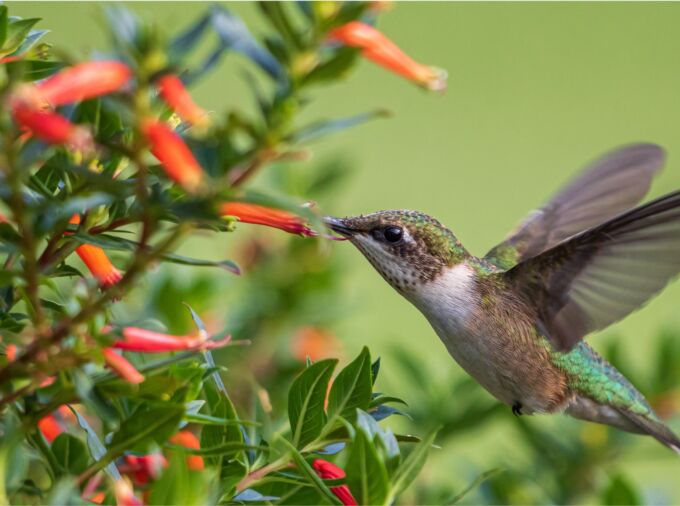
Pennsylvania's Pollinators
What is a pollinator and why do we need them?
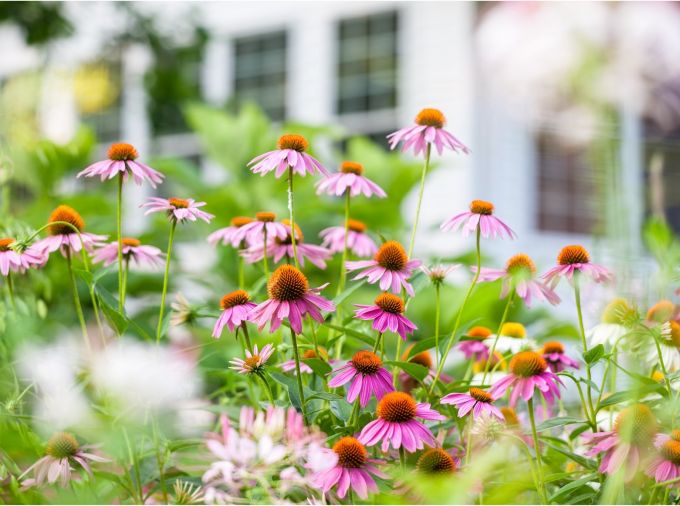
Landscaping for Pollinators
Ways you can help reverse declines in pollinator populations right in your own backyard, including how to certify your property as a Pollinator Friendly Habitat.
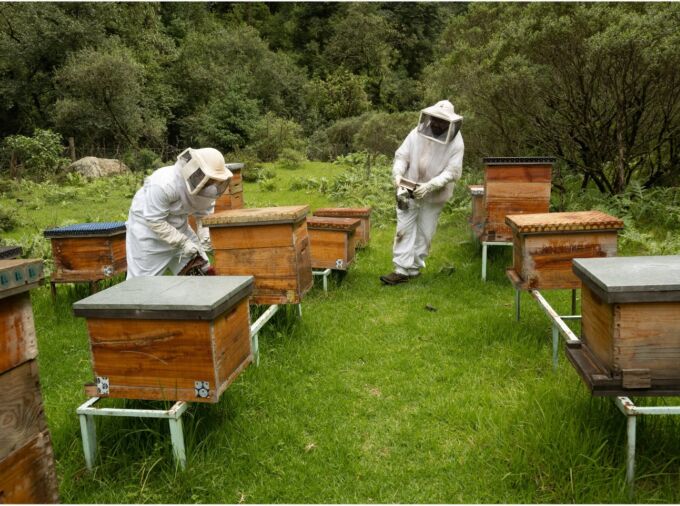
Research
We employ multidisciplinary research approaches committed to studying the factors impacting pollinator health.
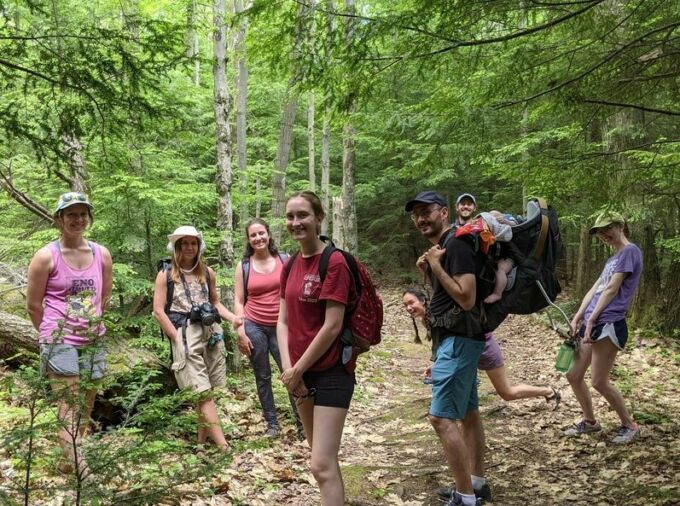
About Us
We are researchers, educators, extension specialists and outreach coordinators spanning multiple departments and colleges.
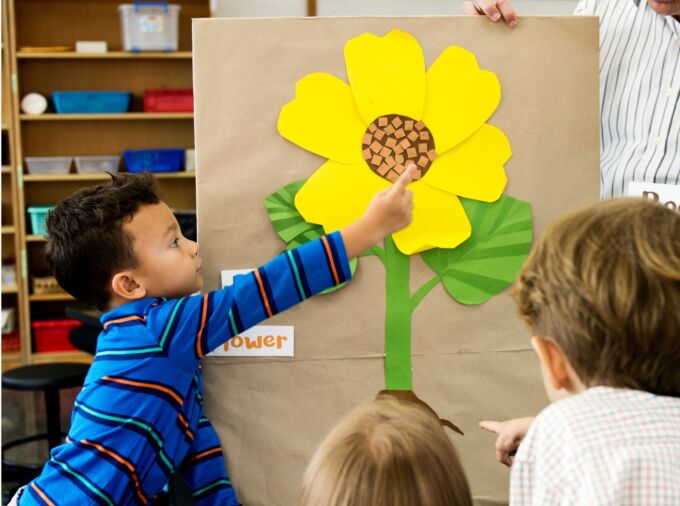
Classroom Materials
A collection of lesson plans and classroom activities all about pollinators!
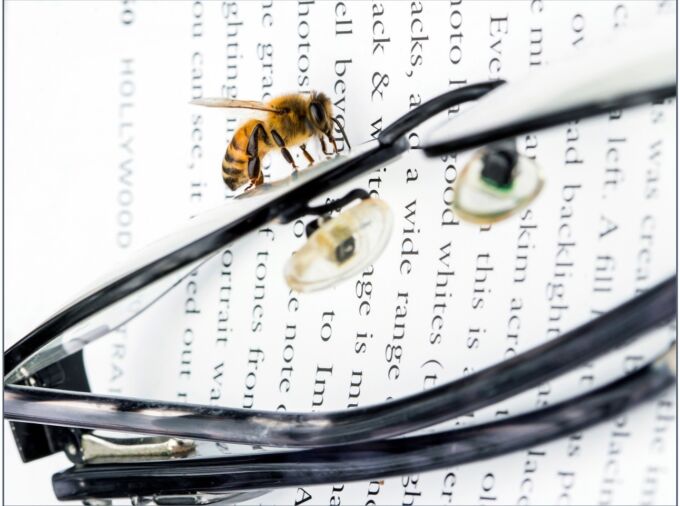
Resource Library
Find information about pollinators by species, resource type, or topic!
News
More News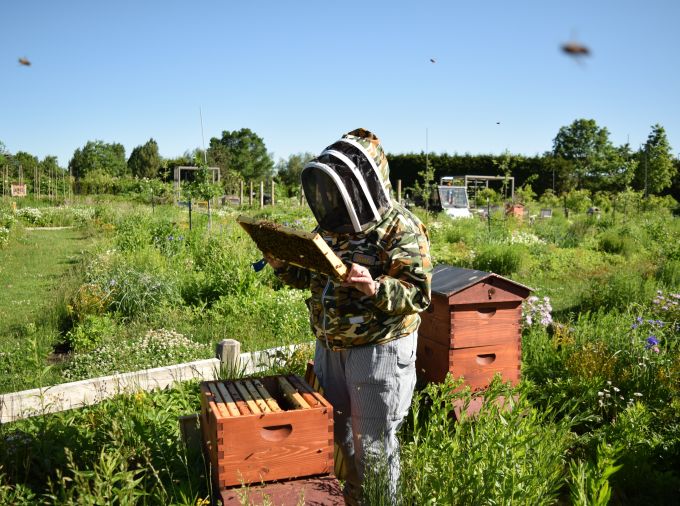
February 12, 2024
Penn State Extension to offer ‘Beekeeping Around the World’ webinar series
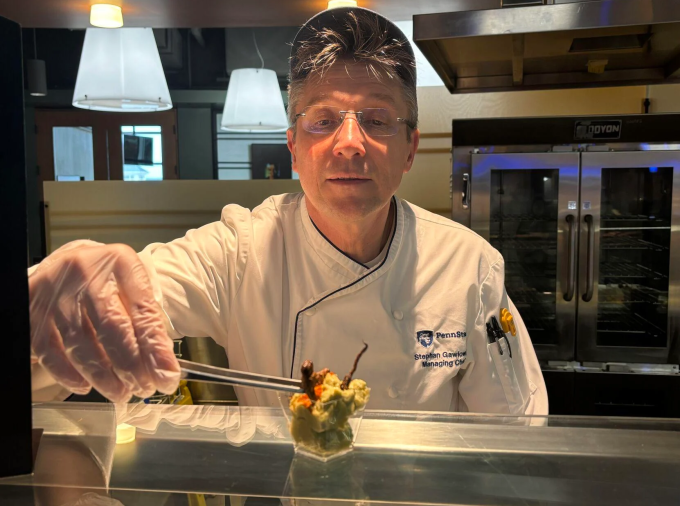
February 09, 2024
Penn State students taste pollen smoothies, honey, and lemon grasshoppers
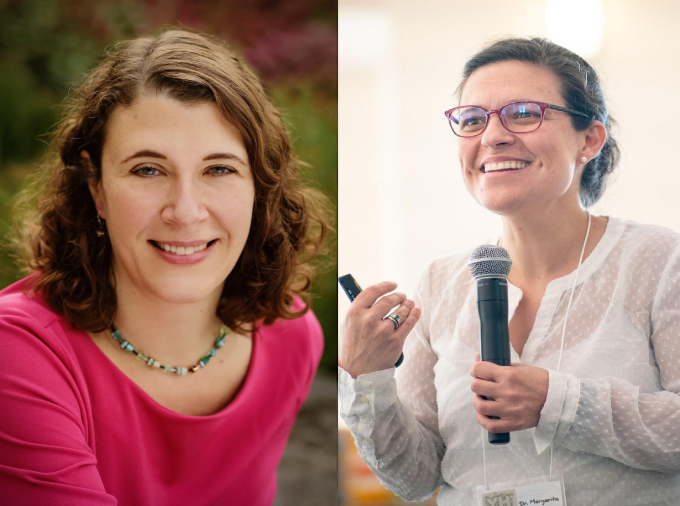
February 02, 2024
Penn State entomologists appointed to national committee on pollinator research
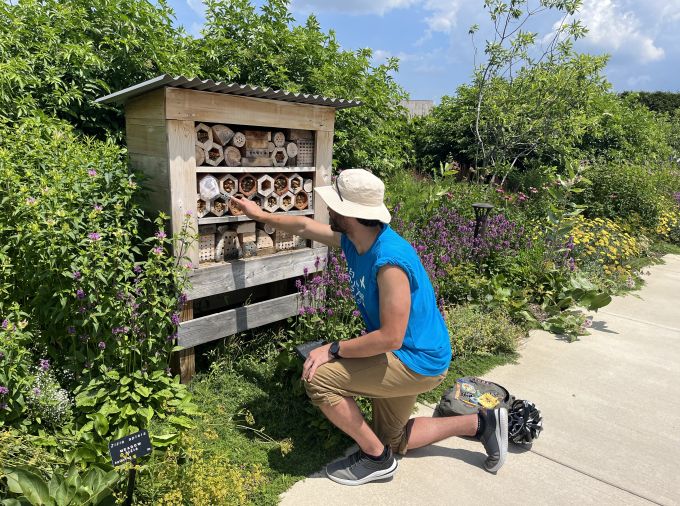
February 01, 2024